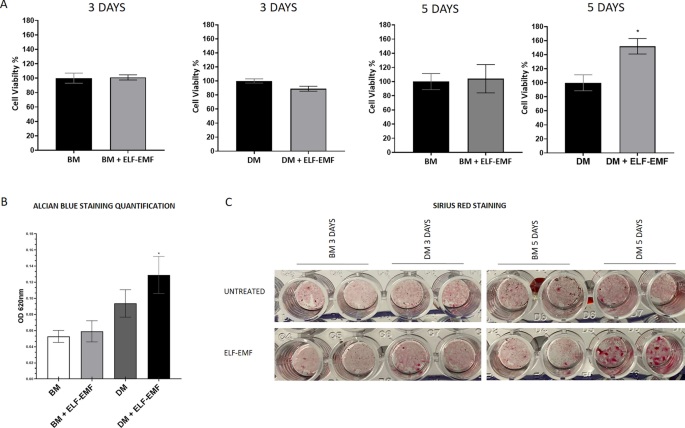
Krishnan, Y. & Grodzinsky, A. J. Cartilage diseases. Matrix Biol. 71–72, 51–69 (2018).
Marcacci, M., Filardo, G. & Kon, E. Treatment of cartilage lesions: What works and why?. Injury 44, S11–S15 (2013).
Becerra, J. et al. Articular cartilage: Structure and regeneration. Tissue Eng. Part B Rev. 16, 617–627 (2010).
Cross, M. et al. The global burden of hip and knee osteoarthritis: Estimates from the global burden of disease 2010 study. Ann. Rheum. Dis. 73, 1323–1330 (2014).
Tong, L. et al. Current understanding of osteoarthritis pathogenesis and relevant new approaches. Bone Res. 10, 1–17 (2022).
McAllister, M. J., Chemaly, M., Eakin, A. J., Gibson, D. S. & McGilligan, V. E. NLRP3 as a potentially novel biomarker for the management of osteoarthritis. Osteoarthr. Cartil. 26, 612–619 (2018).
Maldonado, M. & Nam, J. The role of changes in extracellular matrix of cartilage in the presence of inflammation on the pathology of osteoarthritis. BioMed Res. Int. https://doi.org/10.1155/2013/284873 (2013).
Loo, S. & Wong, N. Advantages and challenges of stem cell therapy for osteoarthritis (Review). Biomed. Rep. 15, 1–12 (2021).
Liu, Y., Wu, J., Zhu, Y. & Han, J. Therapeutic application of mesenchymal stem cells in bone and joint diseases. Clin. Exp. Med. 14, 13–24 (2014).
Discher, D. E., Mooney, D. J. & Zandstra, P. W. Growth factors, matrices, and forces combine. Growth (Lakeland) 324, 1673–1677 (2010).
Wu, S. C., Chang, J. K., Wang, C. K., Wang, G. J. & Ho, M. L. Enhancement of chondrogenesis of human adipose derived stem cells in a hyaluronan-enriched microenvironment. Biomaterials 31, 631–640 (2010).
Robert, A. W., Marcon, B. H., Dallagiovanna, B. & Shigunov, P. Adipogenesis, osteogenesis, and chondrogenesis of human mesenchymal stem/stromal cells: A comparative transcriptome approach. Front. Cell Dev. Biol. 8, 561 (2020).
Jorgensen, C., Noel, D., Apparailly, F. & Sany, J. Stem cells for repair of cartilage and bone: The next challenge in osteoarthritis and rheumatoid arthritis. Ann. Rheum. Dis. 60, 305–309 (2001).
Heng, B. C., Cao, T. & Lee, E. H. Directing stem cell differentiation into the chondrogenic lineage in vitro. Stem Cells 22, 1152–1167 (2004).
Huang, C., Dai, J. & Zhang, X. A. Environmental physical cues determine the lineage specification of mesenchymal stem cells. Biochim. Biophys. Acta BBA Gen. Subj. 1850, 1261–1266 (2015).
Viganò, M. et al. Mesenchymal stem cells as therapeutic target of biophysical stimulation for the treatment of musculoskeletal disorders. J. Orthop. Surg. Res. 11, 163 (2016).
Saliev, T., Begimbetova, D., Masoud, A.-R. & Matkarimov, B. Biological effects of non-ionizing electromagnetic fields: Two sides of a coin. Prog. Biophys. Mol. Biol. 141, 25–36 (2019).
Ross, C. L., Zhou, Y., McCall, C. E., Soker, S. & Criswell, T. L. The use of pulsed electromagnetic field to modulate inflammation and improve tissue regeneration: A review. Bioelectricity 1, 247–259 (2019).
Matthes, R. M. A. F. B. J. H. V. P. V. B. Exposure to static and low frequency electromagnetic fields, biological effects and health consequences (0–100 kHz). Review of the scientific evidence on dosimetry, biological effects, epidemiological observations, and health consequences concerning exposure to static and low frequency electromagnetic fields (0–100 kHz). ETDEWEB (2003).
Polk, C. CRC Handbook of Biological Effects of Electromagnetic Fields (CRC Press, 2019). https://doi.org/10.1201/9781351071017.
Consales, C., Merla, C., Marino, C. & Benassi, B. Electromagnetic fields, oxidative stress, and neurodegeneration. Int. J. Cell Biol. 2012, 1–16 (2012).
Maziarz, A. et al. How electromagnetic fields can influence adult stem cells: Positive and negative impacts. Stem Cell Res. Ther. 7, 54 (2016).
Funk, R. H. W., Monsees, T. & Özkucur, N. Electromagnetic effects – From cell biology to medicine. Prog. Histochem. Cytochem. 43, 177–264 (2009).
Veronesi, F. et al. Experimentally induced cartilage degeneration treated by pulsed electromagnetic field stimulation; An in vitro study on bovine cartilage. BMC Musculoskelet. Disord. 16, 1–9 (2015).
Varani, K. et al. Pulsed electromagnetic field stimulation in osteogenesis and chondrogenesis: Signaling pathways and therapeutic implications. Int. J. Mol. Sci. 22, 1–17 (2021).
Chen, C. H. et al. Electromagnetic fields enhance chondrogenesis of human adipose-derived stem cells in a chondrogenic microenvironment in vitro. J. Appl. Physiol. 114, 647–655 (2013).
Esposito, M. et al. Differentiation of human umbilical cord-derived mesenchymal stem cells, WJ-MSCs, into chondrogenic cells in the presence of pulsed electromagnetic fields. In Vivo (Brooklyn) 27, 495–500 (2013).
Erickson, G. R. et al. Chondrogenic potential of adipose tissue-derived stromal cells in vitro and in vivo. Biochem. Biophys. Res. Commun. 290, 763–769 (2002).
Zhang, L. et al. Chondrogenic differentiation of human mesenchymal stem cells: A comparison between micromass and pellet culture systems. Biotechnol. Lett. 32, 1339–1346 (2010).
Biazzo, A., D’Ambrosi, R., Masia, F., Izzo, V. & Verde, F. Autologous adipose stem cell therapy for knee osteoarthritis: Where are we now?. Phys. Sportsmed. 48, 392–399 (2020).
Yang, C. L. et al. Collagen II from articular cartilage and annulus fibrosus. Eur. J. Biochem. 213, 1297–1302 (1993).
Nah, H., Swoboda, B., Birk, D. E. & Kirsch, T. Type IIA procollagen: Expression in developing chicken limb cartilage and human osteoarthritic articular cartilage. Dev. Dyn. 220, 307–322 (2001).
Lian, C. et al. Collagen type II suppresses articular chondrocyte hypertrophy and osteoarthritis progression by promoting integrin β1−SMAD1 interaction. Bone Res. 7, 8 (2019).
Chen, J.-L., Duan, L., Zhu, W., Xiong, J. & Wang, D. Extracellular matrix production in vitro in cartilage tissue engineering. J. Transl. Med. 12, 88 (2014).
Khodabandehloo, F. et al. Functional differences of Toll-like receptor 4 in osteogenesis, adipogenesis and chondrogenesis in human bone marrow-derived mesenchymal stem cells. J. Cell Mol. Med. 25, 5138–5149 (2021).
Uccelli, A., Moretta, L. & Pistoia, V. Mesenchymal stem cells in health and disease. Nat. Rev. Immunol. 8, 726–736 (2008).
Hunziker, E. B. Articular cartilage repair: Basic science and clinical progress. A review of the current status and prospects. Osteoarthr. Cartil. 10, 432–463 (2002).
Im, GIl. Regeneration of articular cartilage using adipose stem cells. J. Biomed. Mater. Res. A 104, 1830–1844 (2016).
Wu, L., Cai, X., Zhang, S., Karperien, M. & Lin, Y. Regeneration of articular cartilage by adipose tissue derived mesenchymal stem cells: Perspectives from stem cell biology and molecular medicine. J. Cell Physiol. 228, 938–944 (2013).
Pak, J., Lee, J. H., Kartolo, W. A. & Lee, S. H. Cartilage regeneration in human with adipose tissue-derived stem cells: Current status in clinical implications. Biomed. Res. Int. 2016, 1–12 (2016).
Iorio, J. et al. Ultra-low electromagnetic fields application on in vitro cartilage regeneration: A pilot study to improve treatment of osteoarticular diseases. Appl. Sci. 12, 4116 (2022).
Otto, F. et al. Cbfa1, a candidate gene for cleidocranial dysplasia syndrome, is essential for osteoblast differentiation and bone development. Cell 89, 765–771 (1997).
Komori, T. et al. Targeted disruption of Cbfa1 results in a complete lack of bone formation owing to maturational arrest of osteoblasts. Cell 89, 755–764 (1997).
Kim, I. S., Otto, F., Zabel, B. & Mundlos, S. Regulation of chondrocyte differentiation by Cbfa1. Mech. Dev. 80, 159–170 (1999).
Enomoto, H. et al. Cbfa1 is a positive regulatory factor in chondrocyte maturation. J. Biol. Chem. 275, 8695–8702 (2000).
Nishimura, R. et al. Regulation of cartilage development and diseases by transcription factors. J. Bone Metab. 24, 147 (2017).
Aghajanian, P. & Mohan, S. The art of building bone: Emerging role of chondrocyte-to-osteoblast transdifferentiation in endochondral ossification. Bone Res. 6, 19 (2018).
Zheng, Q. et al. Type X collagen gene regulation by Runx2 contributes directly to its hypertrophic chondrocyte-specific expression in vivo. J. Cell Biol. 162, 833–842 (2003).
Inada, M. et al. Maturational disturbance of chondrocytes in Cbfa1-deficient mice. Dev. Dyn. 214, 279–290 (1999).
<a data-track="click" rel="nofollow noopener" data-track-label="10.1002/(SICI)1097-0177(199904)214:43.0.CO;2-W” data-track-action=”article reference” href=”https://doi.org/10.1002%2F%28SICI%291097-0177%28199904%29214%3A4%3C279%3A%3AAID-AJA1%3E3.0.CO%3B2-W” aria-label=”Article reference 50″ data-doi=”10.1002/(SICI)1097-0177(199904)214:43.0.CO;2-W”>Article
CAS
PubMed
Google Scholar
Genç, D. et al. Synovial fluid niche promoted differentiation of dental follicle mesenchymal stem cells toward chondrogenesis in rheumatoid arthritis. Arch. Rheumatol. 37, 94–109 (2022).
Kondo, M. et al. Contribution of the interleukin-6/STAT-3 signaling pathway to chondrogenic differentiation of human mesenchymal stem cells. Arthritis Rheumatol. 67, 1250–1260 (2015).
Liboff, A. R. Ion cyclotron resonance interactions in living systems. SIBE Convegno Nazionale Società Italiana Biofisica Elettrodinamica ATTI IV PAVIA 19, 1–14 (2013).
Liboff, A. R. Geomagnetic cyclotron resonance in living cells. J. Biol. Phys. 13, 99–102 (1985).
Kavand, H., Lintel, H. & Renaud, P. Efficacy of pulsed electromagnetic fields and electromagnetic fields tuned to the ion cyclotron resonance frequency of Ca2+ on chondrogenic differentiation. J. Tissue Eng. Regen. Med. 13, 799–811 (2019).
Schmidt-Rohlfing, B., Silny, J., Woodruff, S. & Gavenis, K. Effects of pulsed and sinusoid electromagnetic fields on human chondrocytes cultivated in a collagen matrix. Rheumatol. Int. 28, 971–977 (2008).
Li, W. et al. Low-frequency electromagnetic fields combined with tissue engineering techniques accelerate intervertebral fusion. Stem Cell Res. Ther. 12, 143 (2021).
Zerillo, L. et al. Antibiofilm and repair activity of ozonated oil in liposome. Microb. Biotechnol. 15, 1422–1433 (2022).
Livak, K. J. & Schmittgen, T. D. Analysis of relative gene expression data using real-time quantitative PCR and the 2-ΔΔCT method. Methods 25, 402–408 (2001).
- SEO Powered Content & PR Distribution. Get Amplified Today.
- PlatoData.Network Vertical Generative Ai. Empower Yourself. Access Here.
- PlatoAiStream. Web3 Intelligence. Knowledge Amplified. Access Here.
- PlatoESG. Carbon, CleanTech, Energy, Environment, Solar, Waste Management. Access Here.
- PlatoHealth. Biotech and Clinical Trials Intelligence. Access Here.
- Source: https://www.nature.com/articles/s41598-024-60846-5