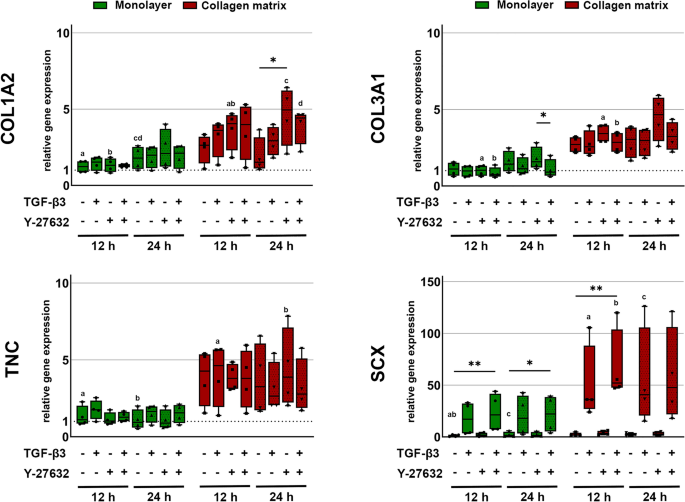
Campbell, B. H., Agarwal, C. & Wang, J.H.-C. TGF-beta1, TGF-beta3, and PGE(2) regulate contraction of human patellar tendon fibroblasts. Biomech. Model. Mechanobiol. 2(4), 239–245. https://doi.org/10.1007/s10237-004-0041-z (2004).
Juneja, S. C., Schwarz, E. M., O’Keefe, R. J. & Awad, H. A. Cellular and molecular factors in flexor tendon repair and adhesions: A histological and gene expression analysis. Connect. Tissue Res. 54(3), 218–226. https://doi.org/10.3109/03008207.2013.787418 (2013).
Shah, M., Foreman, D. M. & Ferguson, M. W. Neutralisation of TGF-beta 1 and TGF-beta 2 or exogenous addition of TGF-beta 3 to cutaneous rat wounds reduces scarring. J. Cell Sci. 108(Pt 3), 985–1002. https://doi.org/10.1242/jcs.108.3.985 (1995).
Maeda, T. et al. Conversion of mechanical force into TGF-β-mediated biochemical signals. Curr. Biol. CB 21(11), 933–941. https://doi.org/10.1016/j.cub.2011.04.007 (2011).
Roth, S. P. et al. Growth factor-mediated tenogenic induction of multipotent mesenchymal stromal cells is altered by the microenvironment of tendon matrix. Cell Transpl. 27(10), 1434–1450. https://doi.org/10.1177/0963689718792203 (2018).
Xu, T., Wu, M., Feng, J., Lin, X. & Gu, Z. RhoA/Rho kinase signaling regulates transforming growth factor-β1-induced chondrogenesis and actin organization of synovium-derived mesenchymal stem cells through interaction with the Smad pathway. Int. J. Mol. Med. 30(5), 1119–1125. https://doi.org/10.3892/ijmm.2012.1107 (2012).
Doll, C. U., Niebert, S. & Burk, J. Mesenchymal stromal cells adapt to chronic tendon disease environment with an initial reduction in matrix remodeling. Int. J. Mol. Sci. 22(23), 12798. https://doi.org/10.3390/ijms222312798 (2021).
Burk, J. et al. Induction of tenogenic differentiation mediated by extracellular tendon matrix and short-term cyclic stretching. Stem Cells Int. https://doi.org/10.1155/2016/7342379 (2016).
Maharam, E. et al. Rho/Rock signal transduction pathway is required for MSC tenogenic differentiation. Bone Res. 3, 15015. https://doi.org/10.1038/boneres.2015.15 (2015).
Xu, B. et al. RhoA/ROCK, cytoskeletal dynamics, and focal adhesion kinase are required for mechanical stretch-induced tenogenic differentiation of human mesenchymal stem cells. J. Cell. Physiol. 227(6), 2722–2729. https://doi.org/10.1002/jcp.23016 (2012).
Hong, S., Lee, J. Y., Hwang, C., Shin, J. H. & Park, Y. Inhibition of rho-associated protein kinase increases the angiogenic potential of mesenchymal stem cell aggregates via paracrine effects. Tissue Eng. Part A 22(3–4), 233–243. https://doi.org/10.1089/ten.TEA.2015.0289 (2016).
Li, Z. et al. Rho kinase inhibitor Y-27632 promotes the differentiation of human bone marrow mesenchymal stem cells into keratinocyte-like cells in xeno-free conditioned medium. Stem Cell Res. Ther. 6(1), 17. https://doi.org/10.1186/s13287-015-0008-2 (2015).
Melzer, M. et al. Rho/ROCK inhibition promotes TGF-β3-induced tenogenic differentiation in mesenchymal stromal cells. Stem Cells Int. 2021, 8284690. https://doi.org/10.1155/2021/8284690 (2021).
Rezaei, H. B., Kamato, D., Ansari, G., Osman, N. & Little, P. J. Cell biology of Smad2/3 linker region phosphorylation in vascular smooth muscle. Clin. Exp. Pharmacol. Physiol. 39(8), 661–667. https://doi.org/10.1111/j.1440-1681.2011.05592.x (2012).
Kretzschmar, M., Doody, J., Timokhina, I. & Massagué, J. A mechanism of repression of TGFbeta/ Smad signaling by oncogenic Ras. Genes Development 13(7), 804–816. https://doi.org/10.1101/gad.13.7.804 (1999).
Matsuzaki, K. Smad phosphoisoform signaling specificity: The right place at the right time. Carcinogenesis 32(11), 1578–1588. https://doi.org/10.1093/carcin/bgr172 (2011).
Kamato, D. et al. Smad linker region phosphorylation is a signalling pathway in its own right and not only a modulator of canonical TGF-β signalling. Cell. Mol. Life Sci. 77(2), 243–251. https://doi.org/10.1007/s00018-019-03266-3 (2020).
Ji, H. et al. Rho/Rock cross-talks with transforming growth factor-β/Smad pathway participates in lung fibroblast-myofibroblast differentiation. Biomed. Rep. 2(6), 787–792. https://doi.org/10.3892/br.2014.323 (2014).
Kamaraju, A. K. & Roberts, A. B. Role of Rho/ROCK and p38 MAP kinase pathways in transforming growth factor-beta-mediated Smad-dependent growth inhibition of human breast carcinoma cells in vivo. J. Biol. Chem. 280(2), 1024–1036. https://doi.org/10.1074/jbc.M403960200 (2005).
Kamato, D. & Little, P. J. Smad2 linker region phosphorylation is an autonomous cell signalling pathway: Implications for multiple disease pathologies. Biomed. Pharmacother. 124, 109854. https://doi.org/10.1016/j.biopha.2020.109854 (2020).
Li, Y. et al. Transforming growth factor-β signalling pathway in tendon healing. Growth Factors (Chur, Switzerland) 40(3–4), 98–107. https://doi.org/10.1080/08977194.2022.2082294 (2022).
Brown, K. A., Pietenpol, J. A. & Moses, H. L. A tale of two proteins: Differential roles and regulation of Smad2 and Smad3 in TGF-beta signaling. J. Cell. Biochem. 101(1), 9–33. https://doi.org/10.1002/jcb.21255 (2007).
Roth, S. P., Burk, J., Brehm, W. & Troillet, A. MSC in tendon and joint disease: The context-sensitive link between targets and therapeutic mechanisms. Front. Bioeng. Biotechnol. 10, 855095. https://doi.org/10.3389/fbioe.2022.855095 (2022).
Huveneers, S. & Danen, E. H. J. Adhesion signalling—Crosstalk between integrins, Src and Rho. J. Cell Sci. 122(Pt 8), 1059–1069. https://doi.org/10.1242/jcs.039446 (2009).
Woods, A., Wang, G. & Beier, F. RhoA/ROCK signaling regulates Sox9 expression and actin organization during chondrogenesis. J. Biol. Chem. 280(12), 11626–11634. https://doi.org/10.1074/jbc.M409158200 (2005).
Browne, J. A., Liu, X., Schnaper, H. W. & Hayashida, T. Serine-204 in the linker region of Smad3 mediates the collagen-I response to TGF-β in a cell phenotype-specific manner. Exp. Cell Res. 319(19), 2928–2937. https://doi.org/10.1016/j.yexcr.2013.07.013 (2013).
Berthet, E. et al. Smad3 binds Scleraxis and Mohawk and regulates tendon matrix organization. J. Orthop. Res. Off. Publ. Orthop. Res. Soc. 31(9), 1475–1483. https://doi.org/10.1002/jor.22382 (2013).
Katzel, E. B. et al. Impact of Smad3 loss of function on scarring and adhesion formation during tendon healing. J. Orthop. Res. Off. Publ. Orthop. Res. Soc. 29(5), 684–693. https://doi.org/10.1002/jor.21235 (2011).
Furumatsu, T., Tsuda, M., Taniguchi, N., Tajima, Y. & Asahara, H. Smad3 induces chondrogenesis through the activation of SOX9 via CREB-binding protein/p300 recruitment. J. Biol. Chem. 280(9), 8343–8350. https://doi.org/10.1074/jbc.M413913200 (2005).
Alliston, T., Choy, L., Ducy, P., Karsenty, G. & Derynck, R. TGF-beta-induced repression of CBFA1 by Smad3 decreases cbfa1 and osteocalcin expression and inhibits osteoblast differentiation. EMBO J. 20(9), 2254–2272. https://doi.org/10.1093/emboj/20.9.2254 (2001).
Sharma, R. I. & Snedeker, J. G. Biochemical and biomechanical gradients for directed bone marrow stromal cell differentiation toward tendon and bone. Biomaterials 31(30), 7695–7704. https://doi.org/10.1016/j.biomaterials.2010.06.046 (2010).
Kuo, C. K. & Tuan, R. S. Mechanoactive tenogenic differentiation of human mesenchymal stem cells. Tissue Eng. Part A 14(10), 1615–1627. https://doi.org/10.1089/ten.tea.2006.0415 (2008).
Kishore, V., Bullock, W., Sun, X., van Dyke, W. S. & Akkus, O. Tenogenic differentiation of human MSCs induced by the topography of electrochemically aligned collagen threads. Biomaterials 33(7), 2137–2144. https://doi.org/10.1016/j.biomaterials.2011.11.066 (2012).
Barsby, T., Bavin, E. P. & Guest, D. J. Three-dimensional culture and transforming growth factor beta3 synergistically promote tenogenic differentiation of equine embryo-derived stem cells. Tissue Eng. Part A 20(19–20), 2604–2613. https://doi.org/10.1089/ten.TEA.2013.0457 (2014).
Khyrul, W. A. K. M., LaLonde, D. P., Brown, M. C., Levinson, H. & Turner, C. E. The integrin-linked kinase regulates cell morphology and motility in a rho-associated kinase-dependent manner. J. Biol. Chem. 279(52), 54131–54139. https://doi.org/10.1074/jbc.M410051200 (2004).
Hong, H., McCullough, C. M. & Stegemann, J. P. The role of ERK signaling in protein hydrogel remodeling by vascular smooth muscle cells. Biomaterials 28(26), 3824–3833. https://doi.org/10.1016/j.biomaterials.2007.05.007 (2007).
Peng, Y. et al. ROCK isoforms differentially modulate cancer cell motility by mechanosensing the substrate stiffness. Acta Biomaterialia 88, 86–101. https://doi.org/10.1016/j.actbio.2019.02.015 (2019).
Yi, B., Xu, Q. & Liu, W. An overview of substrate stiffness guided cellular response and its applications in tissue regeneration. Bioactive Mater. 15, 82–102. https://doi.org/10.1016/j.bioactmat.2021.12.005 (2022).
Franke, K. et al. Topologically defined composites of collagen types I and V as in vitro cell culture scaffolds. Acta Biomaterialia 10(6), 2693–2702 (2014).
Vogel, S. et al. Remodeling of three-dimensional collagen I matrices by human bone marrow stromal cells during osteogenic differentiation in vitro. ACS Appl. Bio Mater. 3(10), 6967–6978 (2020).
Sheng, R., et al. Material Stiffness in Cooperation with Macrophage Paracrine Signals Determines the Tenogenic Differentiation of Mesenchymal Stem Cells. Advanced science (Weinheim, Baden-Wurttemberg, Germany), e2206814. https://doi.org/10.1002/advs.202206814 (2023).
Liu, C. et al. Matrix stiffness regulates the differentiation of tendon-derived stem cells through FAK-ERK1/2 activation. Exp. Cell Res. 373(1–2), 62–70. https://doi.org/10.1016/j.yexcr.2018.08.023 (2018).
Hou, Y. et al. Nonwoven-based gelatin/polycaprolactone membrane loaded with ERK inhibitor U0126 for treatment of tendon defects. Stem Cell Res. Ther. 13(1), 5. https://doi.org/10.1186/s13287-021-02679-x (2022).
Burch, M. L., Zheng, W. & Little, P. J. Smad linker region phosphorylation in the regulation of extracellular matrix synthesis. Cell. Mol. Life Sci. CMLS 68(1), 97–107. https://doi.org/10.1007/s00018-010-0514-4 (2011).
Hough, C., Radu, M. & Doré, J. J. E. Tgf-beta induced Erk phosphorylation of smad linker region regulates smad signaling. PloS One 7(8), e42513. https://doi.org/10.1371/journal.pone.0042513 (2012).
Shih, Y.-R.V., Tseng, K.-F., Lai, H.-Y., Lin, C.-H. & Lee, O. K. Matrix stiffness regulation of integrin-mediated mechanotransduction during osteogenic differentiation of human mesenchymal stem cells. J. Bone Miner. Res. 26(4), 730–738. https://doi.org/10.1002/jbmr.278 (2011).
Schubert, S., Brehm, W., Hillmann, A., Burk, J. (2018) Serum-free human MSC medium supports consistency in human but not in equine adipose-derived multipotent mesenchymal stromal cell culture. Cytometry Part A J. Int. Soc. Analyt. Cytol. 93(1), 60–72. https://doi.org/10.1002/cyto.a.23240.
Dominici, M., et al. (2006) Minimal criteria for defining multipotent mesenchymal stromal cells. The International Society for Cellular Therapy position statement. Cytotherapy. 8(4), 315–317. https://doi.org/10.1080/14653240600855905.
Pfaffl, M. W. A new mathematical model for relative quantification in real-time RT-PCR. Nucleic Acids Res. 29(9), e45. https://doi.org/10.1093/nar/29.9.e45 (2001).
- SEO Powered Content & PR Distribution. Get Amplified Today.
- PlatoData.Network Vertical Generative Ai. Empower Yourself. Access Here.
- PlatoAiStream. Web3 Intelligence. Knowledge Amplified. Access Here.
- PlatoESG. Carbon, CleanTech, Energy, Environment, Solar, Waste Management. Access Here.
- PlatoHealth. Biotech and Clinical Trials Intelligence. Access Here.
- Source: https://www.nature.com/articles/s41598-024-60717-z