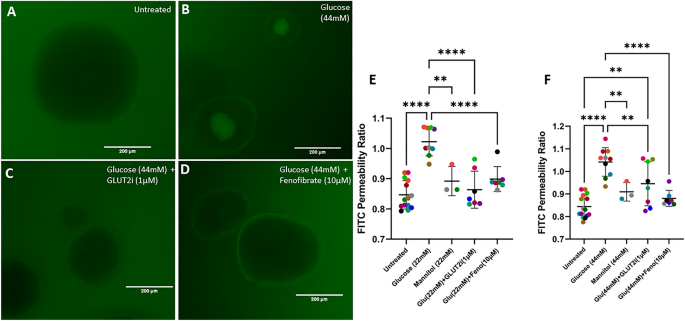
Kaufman, F. R. Type 2 diabetes mellitus in children and youth: a new epidemic. J. Pediatr. Endocrinol. Metab. 15(Suppl 2), 737–744 (2002).
Hospital, B.P., State of Pet Health 2016 Report, in State of Pet Health Report. 2016, Banfield Pet Hospital.
Lederer, R. et al. Frequency of feline diabetes mellitus and breed predisposition in domestic cats in Australia. Vet. J. 179(2), 254–258 (2009).
Lutz, T. A. Mammalian models of diabetes mellitus, with a focus on type 2 diabetes mellitus. Nat. Rev. Endocrinol. 19(6), 350–360 (2023).
Kol, A. et al. Companion animals: Translational scientist’s new best friends. Sci. Transl. Med. 7(308), 308ps21 (2015).
Meldgaard, T. et al. Diabetic enteropathy: from molecule to mechanism-based treatment. J. Diabetes Res. 2018, 3827301 (2018).
Scott-Moncrieff, J. C. Insulin resistance in cats. Vet. Clin. North Am. Small Anim. Pract. 40(2), 241–257 (2010).
Thaiss, C. A. et al. Hyperglycemia drives intestinal barrier dysfunction and risk for enteric infection. Science 359(6382), 1376–1383 (2018).
Barrett, K. E. New ways of thinking about (and teaching about) intestinal epithelial function. Adv. Physiol. Educ. 32(1), 25–34 (2008).
Vancamelbeke, M. & Vermeire, S. The intestinal barrier: a fundamental role in health and disease. Expert Rev. Gastroenterol. Hepatol. 11(9), 821–834 (2017).
Groschwitz, K. R. & Hogan, S. P. Intestinal barrier function: molecular regulation and disease pathogenesis. J. Allergy Clin. Immunol. 124(1), 3–20 (2009).
Citi, S. et al. Cingulin, a new peripheral component of tight junctions. Nature 333(6170), 272–276 (1988).
Furuse, M. et al. Claudin-1 and -2: novel integral membrane proteins localizing at tight junctions with no sequence similarity to occludin. J. Cell Biol. 141(7), 1539–1550 (1998).
Furuse, M. et al. Occludin: a novel integral membrane protein localizing at tight junctions. J. Cell Biol. 123(6 Pt 2), 1777–1788 (1993).
Suzuki, T. Regulation of the intestinal barrier by nutrients: The role of tight junctions. Anim. Sci. J. 91(1), e13357 (2020).
Kuitunen, M. et al. Intestinal permeability to mannitol and lactulose in children with type 1 diabetes with the HLA-DQB1*02 allele. Autoimmunity 35(5), 365–368 (2002).
Mooradian, A. D. et al. Abnormal intestinal permeability to sugars in diabetes mellitus. Diabetologia 29(4), 221–224 (1986).
Amar, J. et al. Involvement of tissue bacteria in the onset of diabetes in humans: evidence for a concept. Diabetologia 54(12), 3055–3061 (2011).
Damci, T. et al. Increased intestinal permeability as a cause of fluctuating postprandial blood glucose levels in Type 1 diabetic patients. Eur. J. Clin. Invest. 33(5), 397–401 (2003).
Selby, A. et al. Pathophysiology, differential diagnosis, and treatment of diabetic Diarrhea. Dig. Dis. Sci. 64(12), 3385–3393 (2019).
Francis, K.L., et al., 1357-P: Diabetic Hyperglycemia impairs intestinal barrier function in the setting of diet-induced obesity. Diabetes 71(Supplement_1) (2022).
Do, M.H., et al., High-glucose or -fructose diet cause changes of the gut microbiota and metabolic disorders in mice without body weight change. Nutrients 10(6) (2018).
Crakes, K. R. et al. Fenofibrate promotes PPARalpha-targeted recovery of the intestinal epithelial barrier at the host-microbe interface in dogs with diabetes mellitus. Sci. Rep. 11(1), 13454 (2021).
Sidhu, G. & Tripp, J. Fenofibrate (StatPearls Publishing, 2023).
Emami, F. et al. Fenofibrate-induced renal dysfunction, yes or no?. J. Res. Med. Sci. 25, 39 (2020).
Keating, G. M. & Croom, K. F. Fenofibrate: a review of its use in primary dyslipidaemia, the metabolic syndrome and type 2 diabetes mellitus. Drugs 67(1), 121–153 (2007).
Knickelbein, J. E., Abbott, A. B. & Chew, E. Y. Fenofibrate and diabetic retinopathy. Curr. Diab. Rep. 16(10), 90 (2016).
Tsunoda, F. et al. Fenofibrate, HDL, and cardiovascular disease in Type-2 diabetes: The DAIS trial. Atherosclerosis 247, 35–39 (2016).
Bajwa, P. J. et al. Fenofibrate inhibits intestinal Cl- secretion by blocking basolateral KCNQ1 K+ channels. Am. J. Physiol. Gastrointest Liver Physiol. 293(6), G1288–G1299 (2007).
Braissant, O. et al. Differential expression of peroxisome proliferator-activated receptors (PPARs): tissue distribution of PPAR-alpha, -beta, and -gamma in the adult rat. Endocrinology 137(1), 354–366 (1996).
Bunger, M. et al. Genome-wide analysis of PPARalpha activation in murine small intestine. Physiol. Genom. 30(2), 192–204 (2007).
Kersten, S. Integrated physiology and systems biology of PPARalpha. Mol. Metab. 3(4), 354–371 (2014).
Azuma, Y. T. et al. PPARalpha contributes to colonic protection in mice with DSS-induced colitis. Int. Immunopharmacol. 10(10), 1261–1267 (2010).
Crakes, K. R. et al. PPARalpha-targeted mitochondrial bioenergetics mediate repair of intestinal barriers at the host-microbe intersection during SIV infection. Proc. Natl. Acad. Sci. USA 116(49), 24819–24829 (2019).
de Vogel-van den Bosch, H. M. et al. PPARalpha-mediated effects of dietary lipids on intestinal barrier gene expression. BMC Genom. 9, 231 (2008).
Zachos, N. C. et al. Human Enteroids/Colonoids and intestinal organoids functionally recapitulate normal intestinal physiology and pathophysiology. J. Biol. Chem. 291(8), 3759–3766 (2016).
Crawford, C. K. et al. Inflammatory cytokines directly disrupt the bovine intestinal epithelial barrier. Sci. Rep. 12(1), 14578 (2022).
Tekes, G., et al., Development of feline ileum- and colon-derived organoids and their potential use to support feline coronavirus infection. Cells. 9(9) (2020).
Miyoshi, H. & Stappenbeck, T. S. In vitro expansion and genetic modification of gastrointestinal stem cells in spheroid culture. Nat. Protoc. 8(12), 2471–2482 (2013).
Powell, R. H. & Behnke, M. S. WRN conditioned media is sufficient for in vitro propagation of intestinal organoids from large farm and small companion animals. Biol. Open 6(5), 698–705 (2017).
Schmidl, S. et al. Identification of new GLUT2-selective inhibitors through in silico ligand screening and validation in eukaryotic expression systems. Sci. Rep. 11(1), 13751 (2021).
Grosheva, I. et al. High-throughput screen identifies host and microbiota regulators of intestinal barrier function. Gastroenterology 159(5), 1807–1823 (2020).
Tokuda, S., Higashi, T. & Furuse, M. ZO-1 knockout by TALEN-mediated gene targeting in MDCK cells: involvement of ZO-1 in the regulation of cytoskeleton and cell shape. PLoS One 9(8), e104994 (2014).
El-Remessy, A. B. et al. High glucose-induced tyrosine nitration in endothelial cells: role of eNOS uncoupling and aldose reductase activation. Invest. Ophthalmol. Vis. Sci. 44(7), 3135–3143 (2003).
Young, T. K., Lee, S. C. & Tai, L. N. Mannitol absorption and excretion in uremic patients regularly treated with gastrointestinal perfusion. Nephron 25(3), 112–116 (1980).
Mullin, J. M. et al. Increased tight junction permeability can result from protein kinase C activation/translocation and act as a tumor promotional event in epithelial cancers. Ann. N. Y. Acad. Sci. 915, 231–236 (2000).
Rosson, D. et al. Protein kinase C-alpha activity modulates transepithelial permeability and cell junctions in the LLC-PK1 epithelial cell line. J. Biol. Chem. 272(23), 14950–14953 (1997).
Ali, F. Y. et al. Antiplatelet actions of statins and fibrates are mediated by PPARs. Arterioscler. Thromb. Vasc. Biol. 29(5), 706–711 (2009).
Turner, J. R. et al. Physiological regulation of epithelial tight junctions is associated with myosin light-chain phosphorylation. Am. J. Physiol. 273(4), C1378–C1385 (1997).
Thorens, B. GLUT2, glucose sensing and glucose homeostasis. Diabetologia 58(2), 221–232 (2015).
Filippello, A., et al. High glucose exposure impairs l-cell differentiation in intestinal organoids: Molecular mechanisms and clinical implications. Int. J. Mol. Sci. 22(13) (2021).
Forcheron, F. et al. Mechanisms of the triglyceride- and cholesterol-lowering effect of fenofibrate in hyperlipidemic type 2 diabetic patients. Diabetes 51(12), 3486–3491 (2002).
Serisier, S. et al. Fenofibrate lowers lipid parameters in obese dogs. J. Nutr. 136(7 Suppl), 2037S-2040S (2006).
Mazzon, E. & Cuzzocrea, S. Absence of functional peroxisome proliferator-activated receptor-alpha enhanced ileum permeability during experimental colitis. Shock 28(2), 192–201 (2007).
Mazzon, E. & Cuzzocrea, S. Role of TNF-alpha in ileum tight junction alteration in mouse model of restraint stress. Am. J. Physiol. Gastrointest Liver Physiol. 294(5), G1268–G1280 (2008).
Grabacka, M., et al., The role of PPAR alpha in the modulation of innate immunity. Int. J. Mol. Sci. 22(19) (2021).
Wang, X. et al. Fenofibrate ameliorated systemic and retinal inflammation and modulated gut microbiota in high-fat diet-induced mice. Front. Cell Infect. Microbiol. 12, 839592 (2022).
Unsworth, A. J., Flora, G. D. & Gibbins, J. M. Non-genomic effects of nuclear receptors: insights from the anucleate platelet. Cardiovasc. Res. 114(5), 645–655 (2018).
Nakashima, S. Protein kinase C alpha (PKC alpha): regulation and biological function. J. Biochem. 132(5), 669–675 (2002).
Song, J. C., Rangachari, P. K. & Matthews, J. B. Opposing effects of PKCalpha and PKCepsilon on basolateral membrane dynamics in intestinal epithelia. Am. J. Physiol. Cell Physiol. 283(5), C1548–C1556 (2002).
Koya, D. & King, G. L. Protein kinase C activation and the development of diabetic complications. Diabetes 47(6), 859–866 (1998).
Lee, T. S. et al. Activation of protein kinase C by elevation of glucose concentration: proposal for a mechanism in the development of diabetic vascular complications. Proc. Natl. Acad. Sci. USA 86(13), 5141–5145 (1989).
Alt, N. et al. Day-to-day variability of blood glucose concentration curves generated at home in cats with diabetes mellitus. J. Am. Vet. Med. Assoc. 230(7), 1011–1017 (2007).
Gottlieb, S. & Rand, J. Managing feline diabetes: current perspectives. Vet. Med. (Auckl) 9, 33–42 (2018).
- SEO Powered Content & PR Distribution. Get Amplified Today.
- PlatoData.Network Vertical Generative Ai. Empower Yourself. Access Here.
- PlatoAiStream. Web3 Intelligence. Knowledge Amplified. Access Here.
- PlatoESG. Carbon, CleanTech, Energy, Environment, Solar, Waste Management. Access Here.
- PlatoHealth. Biotech and Clinical Trials Intelligence. Access Here.
- Source: https://www.nature.com/articles/s41598-023-49874-9